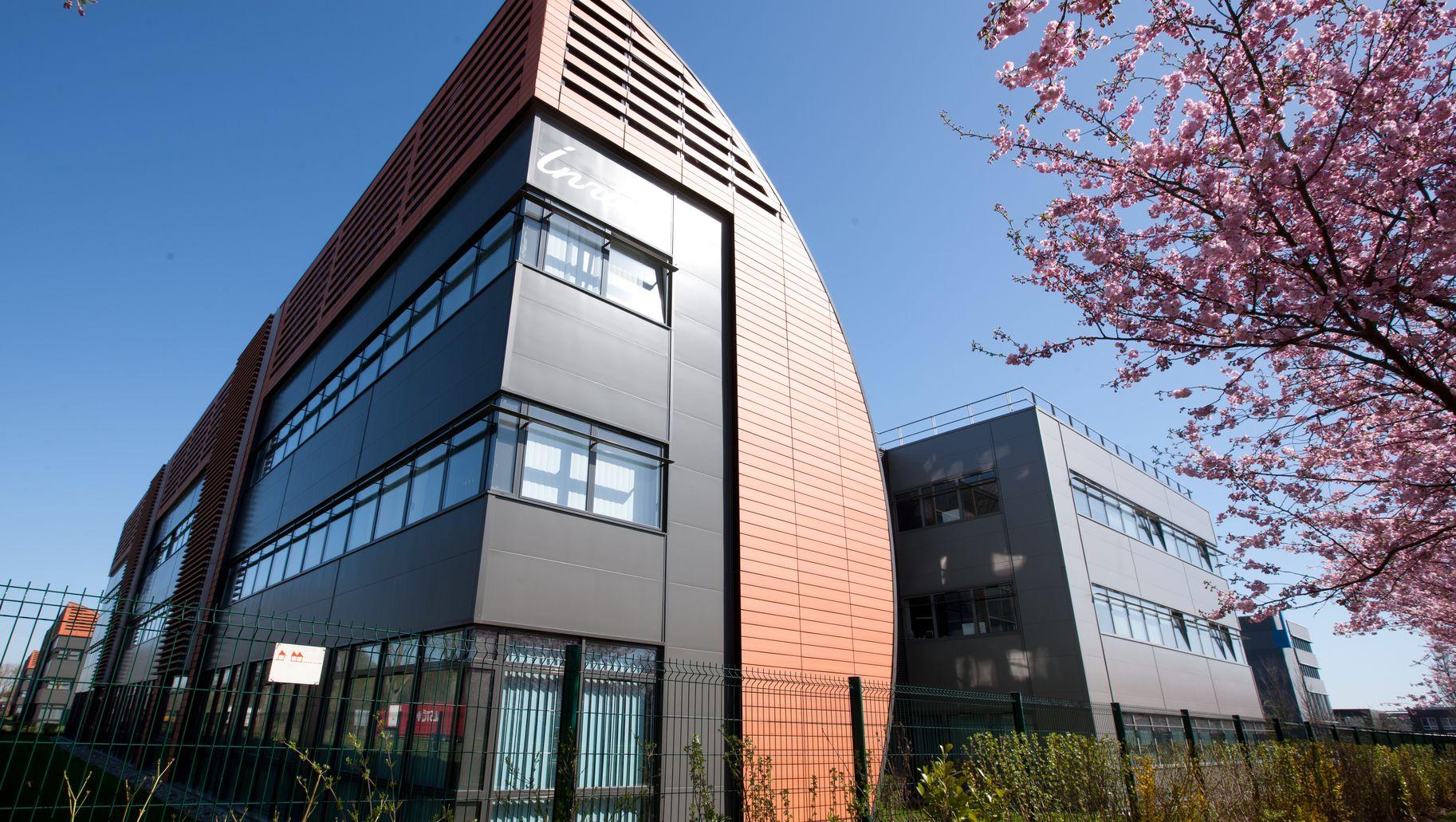
Centre Inria
de l'Université de Lille
de l'Université de Lille
Le Centre Inria de l’Université de Lille compte 15 équipes-projets interagissant en lien étroit avec les laboratoires et les établissements de recherche et d'enseignement supérieur locaux (Université de Lille, CNRS, Inserm, Centrale, Polytech Lille, CHU de Lille…) ainsi qu’avec les acteurs économiques du territoire. Il abrite 365 scientifiques et personnels d’appui à la recherche et à l’innovation, formant ensemble une force vive interculturelle riche de 30 nationalités différentes.
Sciences des données, intelligence artificielle : le traitement, l’équité, l’analyse, la valorisation et l’optimisation des données en grand nombre.
Génie logiciel : la sécurité, l’évolution, l’interopérabilité et l’efficacité énergétique des logiciels et systèmes distribués.
Systèmes cyber-physiques : la conception et le contrôle en robotique souple, déformable et bio-inspirée ; Les réseaux de capteurs et leurs infrastructures (filtrage, transmission, sureté, efficacité énergétique).
Deux thématiques transverses fortes au centre Inria de l’Université de Lille :
Santé numérique : structuration, traitement, interopérabilité et confidentialité des données des hôpitaux pour une médecine personnalisée.
Sobriété numérique : développement d’un numérique intégrant la contrainte énergétique et la réduction de l’impact environnemental.
Scientifiques, ingénieur.e.s, personnels d’appui à la recherche : rejoignez un environnement professionnel stimulant.
Tous les ans, Inria recrute des stagiaires, doctorant.e.s, post-doctorant.e.s, chargé.e.s de recherche et des directeurs ou directrices de recherche, par voie de concours ou sur contrat.
Inria propose un environnement unique à de nombreux talents dans le domaine du développement technologique et logiciel. Le métier d’Ingénieur de recherche est l’acteur essentiel pour accompagner et garantir la qualité de la production de savoirs et de valeur des équipes de recherche.
Inria fait appel à des talents dans plus d’une quarantaine de métiers d'appui à la recherche afin de réaliser son ambition des ressources humaines à l’assistanat administratif et la gestion financière en passant par la communication.
Vous souhaitez visiter Interface ? Il est situé dans le bâtiment Inria d'EuraTechnologies. Les visites se font sur rendez-vous uniquement en écrivant à interface-lille@inria.fr
Adresse à la Haute-Borne
Parc scientifique de la Haute-Borne
40, avenue Halley - Bât A - Park Plaza
59650 Villeneuve d'Ascq - France
Adresse à EuraTechnologies
Bâtiment Place Inria
172 avenue de Bretagne
59 000 Lille
Contacts